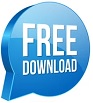
In inorganic oxide perovskites, efficiencies reaching 20% may be achievable, based on the detailed balance limit and spectroscopically limited maximum efficiency, reinstating this class of materials as potentially viable commercial solar absorbers. The efficiency achieved is the highest (along with Cu 2O) for oxide-based photovoltaic absorbers. The band gap of Bi 2FeCrO 6 thin films was reduced to 1.4 eV through Fe/Cr cation disorder and strain relaxation, and 8.1%-efficient photovoltaics were achieved, although processing temperatures were relatively high, at 600 ☌. Recently, a breakthrough in the efficiency of oxide perovskite photovoltaics was achieved. By contrast, oxide perovskites are more environmentally and thermally stable, but require high processing temperatures. But while lead-halide perovskites have the advantages of processability at low temperature (with typical processing temperatures of 100 ☌) and exceptional optoelectronic properties, they are limited by their composition of toxic lead and limited environmental and thermal stability without encapsulation. The learning rate demonstrated is higher than in other commercialized absorbers, and is due to a variety of factors, including narrow band gaps (e.g., 1.55 eV for MAPbI 3), high absorption coefficients ≈10 5 cm –1, long diffusion lengths >1 μm, defect tolerance, low Urbach energies, and high photoluminescence quantum efficiencies (PLQEs). From an initially reported PV efficiency of 3.8% (using methylammonium lead iodide, or MAPbI 3) in 2009, LHP photovoltaics have achieved a certified efficiency of 25.5% in 2020. More recently, the performance of oxide perovskite solar absorbers was rapidly superseded by lead-halide perovskites (LHPs). They have also found applications in photovoltaics (PVs), but the wide band gap of typical perovskite oxides >2.5 eV has historically limited efficiencies to below 1.25%. Historically, oxide perovskites have been used in ferroelectric, piezoelectric and pyroelectric applications. There is therefore wide flexibility in the composition of oxide perovskites, and only a fraction of all possible compounds has been synthesized so far. Furthermore, a variety of A- and B-site formal charge combinations can be used, provided they equal to +6 for single oxide perovskites and +12 for double oxide perovskites. Beyond these single perovskites, two different A-site cations or B-site cations with different formal charges can be used, giving rise to double perovskites with the formulae A′A″B 2O 6 or A 2B′B″O 6, respectively. Historically, perovskite research has focused on oxide compounds, such as CaTiO 3 (discovered by Rose in 1839 and named after Lev Perovskiy), BaTiO 3, PbTiO 3, SrTiO 3, BiFeO 3, and others. Perovskites were first discovered nearly 200 years ago in a chlorite-rich skarn by Gustav Rose, but did not rise to prominence until the 1940s when their suitability for electronic applications was realized. In the perovskite crystal structure, the B-site cation is octahedrally coordinated, and the n – octahedra form a corner-sharing network. Perovskite refers to a family of compounds with the chemical formula ABX 3.
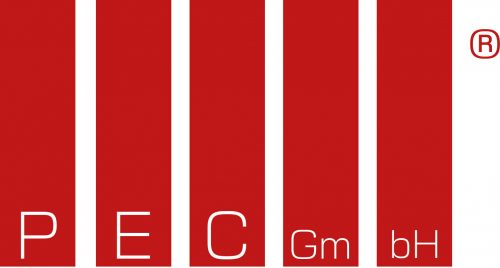
It is finished with a discussion of the implications on the classes of materials future efforts should focus on, as well as the key questions that need to be addressed. Particular emphasis is placed on carrier/exciton-phonon coupling, which plays a significant role in the materials considered, owing to their soft lattices and composition of heavy elements, and becomes more prominent as dimensionality is reduced.
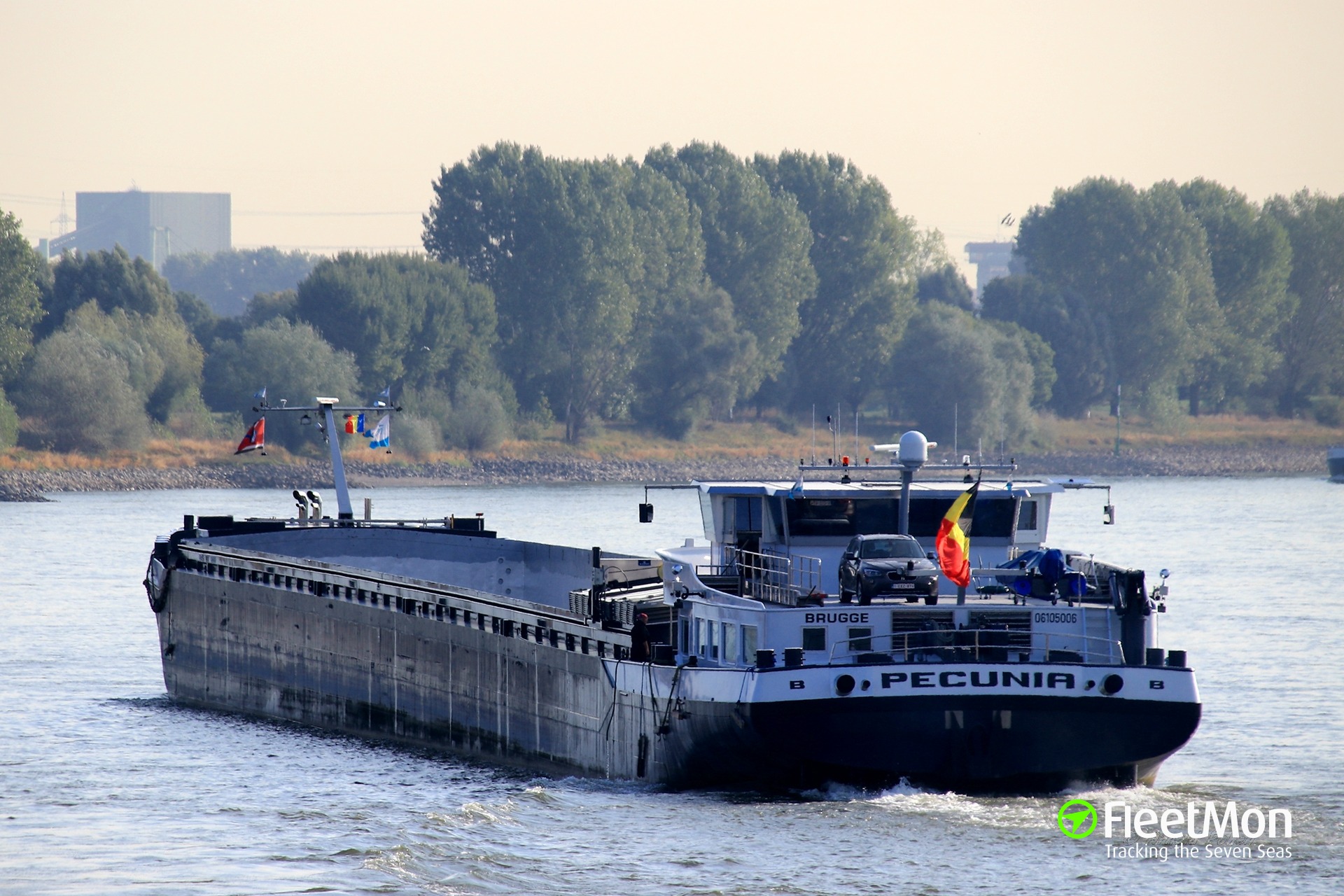
Insights into how dimensionality influences the band gap, stability, charge-carrier transport, recombination processes and defect tolerance of the materials, and the impact these parameters have on device performance are brought forward. This review article examines the role of structural and electronic dimensionality, as well as form factor, in oxide and halide perovskites, and in lead-free alternatives to halide perovskites. One of the many important factors underpinning these developments is an understanding of the role of dimensionality on the optoelectronic properties and, consequently, on the performance of the materials in photovoltaics and LEDs. Halide perovskite semiconductors have risen to prominence in photovoltaics and light-emitting diodes (LEDs), but traditional oxide perovskites, which overcome the stability limitations of their halide counterparts, have also recently witnessed a rise in potential as solar absorbers.
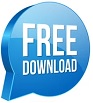